
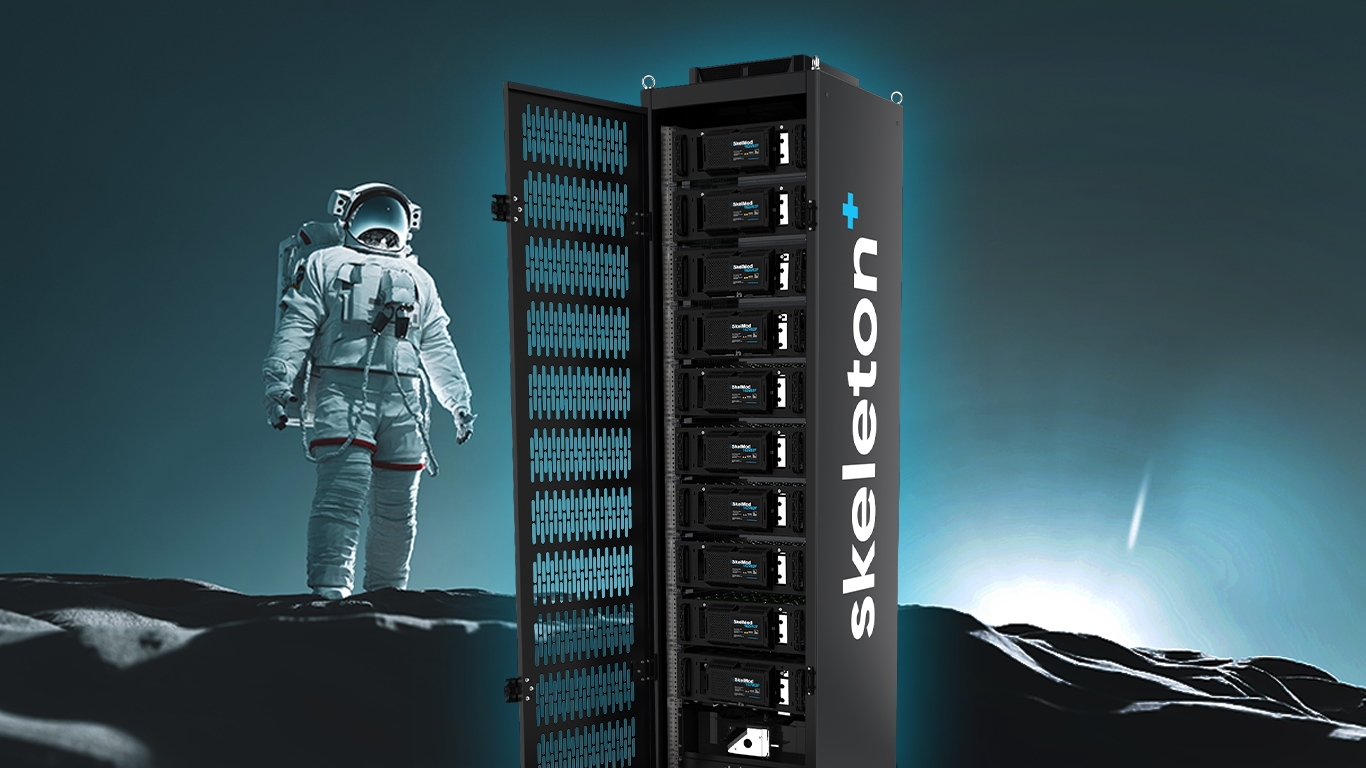
In this post, we delve into what a supercapacitor is. This guide, crafted for audiences ranging from inquisitive youngsters to seasoned experts, offers insights into high-power energy storage that combines speed with sustainability.
Level 1💡 : Exploring the Basics
Imagine you have a super-fast bike that can go from really slow to super fast in just a few seconds. A supercapacitor is like that bike, but for energy. It's made of special stuff, mostly from something like charcoal but much cooler because it has superpowers to hold and let go of energy very, very quickly. Imagine if you could charge your toy in a blink of an eye—that's what supercapacitors can do for some big gadgets! They're special because they can take in and give out energy faster than anything else we know.
Supercapacitors are like the heavy-duty champions of the energy world. They help keep things running smoothly, like making sure the internet stays on even if the power goes out, so you can still search for things on Google. They also help the big machines at the hospital, like the one that takes pictures of your friend's head if they get hurt while biking.
So, whenever something needs a quick energy boost, like a superhero coming to the rescue, supercapacitors are there to save the day.
Level 2 💡💡: A Closer Look
Supercapacitors, also known as ultracapacitors, represent a leap forward in energy storage technology, bridging the gap between conventional batteries and capacitors with their remarkable capability for rapid energy transfer. These devices are engineered from advanced carbon-based materials which are selected for their high surface area and electrical conductivity. This unique composition enables supercapacitors to absorb and release energy at speeds unattainable by traditional batteries.
The core of a supercapacitor's functionality lies in its ability to store electrical charge not through chemical reactions, as batteries do, but electrostatically—across an electric double layer. This phenomenon occurs at the interface between the electrode material and an electrolyte solution, where charge separation results in rapid energy storage and release capabilities. The efficiency of this process is further enhanced by the porous nature of the electrode materials, which significantly increases the effective surface area for charge storage.
One of the pivotal advantages of supercapacitors is their sustainability profile. The reliance on carbon-based materials, which are more abundant and have a lower environmental impact than the metals used in many batteries (such as lithium, cobalt, and nickel), positions supercapacitors as a more eco-friendly alternative for energy storage. This aspect is particularly relevant in the context of global efforts to reduce dependency on finite resources and minimize ecological footprints.
In practical terms, supercapacitors shine in scenarios that demand quick bursts of power. Their high power density makes them ideal for applications ranging from emergency power backups that ensure uninterrupted operation of critical systems, to smoothing out the energy supply from renewable sources like solar panels and wind turbines, addressing the inherent intermittency of these energy sources. Moreover, their durability and ability to withstand thousands of charge-discharge cycles without significant degradation make them a robust component in the energy storage landscape.
The integration of supercapacitors into existing and future energy systems holds the promise of enhancing efficiency and reliability, from grid stabilization efforts to the development of more responsive and sustainable technology solutions. As research continues to push the boundaries of material science and electrochemistry, supercapacitors are poised to play an increasingly significant role in our energy infrastructure, heralding a future where rapid, efficient, and sustainable energy storage is not just an aspiration but a reality.
Level 3 💡💡💡: Deep Dive
Supercapacitors, also referred to as ultracapacitors and electric double-layer capacitors (EDLC), are distinguished by their unique electrochemical properties, which allow for exceptionally high power densities compared to conventional energy storage devices. This heightened performance is primarily attributed to their operational mechanism, which involves a combination of electrostatic storage in the electric double-layer (EDL) and faradaic reactions contributing to pseudocapacitance.
The EDL capacitance arises at the interface between the electrode material, typically comprised of highly porous carbon-based structures, and the electrolyte. Here, charge separation occurs without involving any chemical reactions. The high surface area of the carbon-based electrodes facilitates the formation of a dense EDL, minimizing the effective distance for ion movement during charge and discharge processes. This, coupled with the rapid mobility of ions in the electrolyte, enables supercapacitors to achieve rapid energy transfer rates, surpassing the limitations posed by diffusion processes within solid electrodes characteristic of traditional batteries.
In addition to the EDL capacitance, pseudocapacitance contributes significantly to the overall capacitance of supercapacitors. Pseudocapacitance arises from reversible redox reactions, intercalation, or electrosorption processes occurring at or near the electrode surface. This dynamic behavior allows for additional charge storage beyond what is achievable through purely electrostatic means, further enhancing the energy storage capacity and performance of supercapacitors.
The choice of materials for supercapacitor construction is paramount in optimizing their electrochemical performance. Carbon-based materials are preferred for their high surface area and conductivity, which are directly proportional to the capacitance value. Additionally, the electrolyte composition plays a crucial role in determining the operational voltage and performance stability of supercapacitors. For instance, Skeleton's supercapacitors utilize an electrolyte comprising organic conductive salts dissolved in acetonitrile. This blend offers high ionic conductivity and low viscosity, facilitating rapid ion transport and double-layer formation, thereby enhancing high-power performance.
From a technical standpoint, the operational voltage of supercapacitors is limited by the electrochemical stability window of the electrolyte. Careful selection and design of electrolyte components are necessary to ensure optimal performance and longevity of supercapacitors in various applications.
In practical terms, the high power density, rapid charge-discharge cycling capability, and long cycle life of supercapacitors make them well-suited for a wide range of applications requiring quick energy transactions. These include regenerative braking systems in transportation, power smoothing in renewable energy installations, load leveling in power grids, and short-term energy storage in pulsed power applications.
In summary, the unparalleled electrochemical and physical properties of supercapacitors, driven by advancements in materials science and electrolyte engineering, position them as promising candidates for addressing the escalating demand for efficient energy storage solutions in diverse industrial sectors. Continued research and innovation in this field hold the key to further enhancing the performance and applicability of supercapacitors in future energy systems.